Quantum mechanics: how the future might influence the past
In 2022, the physics Nobel prize was awarded for experimental work showing that the quantum world must break some of our fundamental intuitions about how the universe works

Estimated reading time: 13 minutes
Huw Price, University of Cambridge and Ken Wharton, San José State University
Many look at those experiments and conclude that they challenge “locality” — the intuition that distant objects need a physical mediator to interact. And indeed, a mysterious connection between distant particles would be one way to explain these experimental results.
Others instead think the experiments challenge “realism” — the intuition that there’s an objective state of affairs underlying our experience. After all, the experiments are only difficult to explain if our measurements are thought to correspond to something real. Either way, many physicists agree about what’s been called “the death by experiment” of local realism.
But what if both of these intuitions can be saved, at the expense of a third? A growing group of experts think that we should abandon instead the assumption that present actions can’t affect past events. Called “retrocausality”, this option claims to rescue both locality and realism.
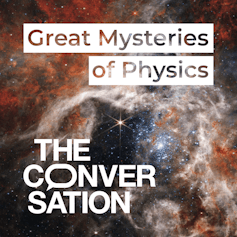
This is article is accompanied by a podcast series called Great Mysteries of Physics which uncovers the greatest mysteries facing physicists today – and discusses the radical proposals for solving them.
Causation
What is causation anyway? Let’s start with the line everyone knows: correlation is not causation. Some correlations are causation, but not all. What’s the difference?
Consider two examples. (1) There’s a correlation between a barometer needle and the weather – that’s why we learn about the weather by looking at the barometer. But no one thinks that the barometer needle is causing the weather. (2) Drinking strong coffee is correlated with a raised heart rate. Here it seems right to say that the first is causing the second.
The difference is that if we “wiggle” the barometer needle, we won’t change the weather. The weather and the barometer needle are both controlled by a third thing, the atmospheric pressure – that’s why they are correlated. When we control the needle ourselves, we break the link to the air pressure, and the correlation goes away.
But if we intervene to change someone’s coffee consumption, we’ll usually change their heart rate, too. Causal correlations are those that still hold when we wiggle one of the variables.
These days, the science of looking for these robust correlations is called “causal discovery”. It’s a big name for a simple idea: finding out what else changes when we wiggle things around us.
In ordinary life, we usually take for granted that the effects of a wiggle are going to show up later than the wiggle itself. This is such a natural assumption that we don’t notice that we’re making it.
But nothing in the scientific method requires this to happen, and it is easily abandoned in fantasy fiction. Similarly in some religions, we pray that our loved ones are among the survivors of yesterday’s shipwreck, say. We’re imagining that something we do now can affect something in the past. That’s retrocausality.
Quantum retrocausality
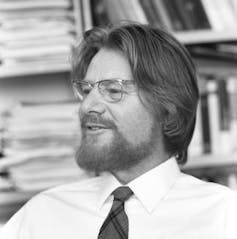
The quantum threat to locality (that distant objects need a physical mediator to interact) stems from an argument by the Northern Ireland physicist John Bell in the 1960s. Bell considered experiments in which two hypothetical physicists, Alice and Bob, each receive particles from a common source. Each chooses one of several measurement settings, and then records a measurement outcome. Repeated many times, the experiment generates a list of results.
Bell realised that quantum mechanics predicts that there will be strange correlations (now confirmed) in this data. They seemed to imply that Alice’s choice of setting has a subtle “nonlocal” influence on Bob’s outcome, and vice versa – even though Alice and Bob might be light years apart. Bell’s argument is said to pose a threat to Albert Einstein’s theory of special relativity, which is an essential part of modern physics.
But that’s because Bell assumed that quantum particles don’t know what measurements they are going to encounter in the future. Retrocausal models propose that Alice’s and Bob’s measurement choices affect the particles back at the source. This can explain the strange correlations, without breaking special relativity.
In recent work, we’ve proposed a simple mechanism for the strange correlation – it involves a familiar statistical phenomenon called Berkson’s bias (see our popular summary here).
There’s now a thriving group of scholars who work on quantum retrocausality. But it’s still invisible to some experts in the wider field. It gets confused for a different view called “superdeterminism”.
Superdeterminism
Superdeterminism agrees with retrocausality that measurement choices and the underlying properties of the particles are somehow correlated.
But superdeterminism treats it like the correlation between the weather and the barometer needle. It assumes there’s some mysterious third thing – a “superdeterminer” – that controls and correlates both our choices and the particles, the way atmospheric pressure controls both the weather and the barometer.
So superdeterminism denies that measurement choices are things we are free to wiggle at will, they are predetermined. Free wiggles would break the correlation, just as in the barometer case. Critics object that superdeterminism thus undercuts core assumptions necessary to undertake scientific experiments. They also say that it means denying free will, because something is controlling both the measurement choices and particles.
These objections don’t apply to retrocausality. Retrocausalists do scientific causal discovery in the usual free, wiggly way. We say it is folk who dismiss retrocausality who are forgetting the scientific method, if they refuse to follow the evidence where it leads.
Evidence
What is the evidence for retrocausality? Critics ask for experimental evidence, but that’s the easy bit: the relevant experiments just won a Nobel Prize. The tricky part is showing that retrocausality gives the best explanation of these results.
We’ve mentioned the potential to remove the threat to Einstein’s special relativity. That’s a pretty big hint, in our view, and it’s surprising it has taken so long to explore it. The confusion with superdeterminism seems mainly to blame.
In addition, we and others have argued that retrocausality makes better sense of the fact that the microworld of particles doesn’t care about the difference between past and future.
We don’t mean that it is all plain sailing. The biggest worry about retrocausation is the possibility of sending signals to the past, opening the door to the paradoxes of time travel. But to make a paradox, the effect in the past has to be measured. If our young grandmother can’t read our advice to avoid marrying grandpa, meaning we wouldn’t come to exist, there’s no paradox. And in the quantum case, it’s well known that we can never measure everything at once.
Still, there’s work to do in devising concrete retrocausal models that enforce this restriction that you can’t measure everything at once. So we’ll close with a cautious conclusion. At this stage, it’s retrocausality that has the wind in its sails, so hull down towards the biggest prize of all: saving locality and realism from “death by experiment”.
Huw Price, Emeritus Fellow, Trinity College, University of Cambridge and Ken Wharton, Professor of Physics and Astronomy, San José State University
This article is republished from The Conversation under a Creative Commons license. Read the original article.
What's Your Reaction?




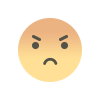
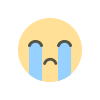
